In recent years, the scientific research interest on nanomaterials has focused on the controlled growth of metal and semiconductor nanoparticles (NPs) and their multidisciplinary applications in very different fields, which range from nano-electronics to nano- photonics and nano-structured photovoltaic devices[1] [2] [3] or nanomaterials for pharmacology and medicine.
Metal and semiconductor nanoparticles have been grown by different chemical and physical methods including Physical Vapor Deposition, Chemical Reactions, and Laser Ablation Synthesis in Solution (LASiS). The last technique is the most used due its low cost and green chemistry characteristics[4]. However, many aspects of growth processes are still unknown and require more detailed studies.
In LASiS the NPs are obtained by irradiation of a pure metal or semiconductor with a pulsed laser (YAG or Kr-F). Laser and material parameters, such as bulk target, solvent and solutes, system temperature and pressure, laser wavelength, duration of irradiation strongly affect the shape and dimensions of the produced nanoparticles. The change of one or several of these parameters can result in the production of very different NPs in both sizes and dimensions. In this scenario, the ability to precisely control the characteristics of NPs by choosing appropriate laser and material parameters is strategic and requires a detailed understanding of the LASiS process4. When a laser beam impacts on a target surface a plume of ablated materials expands in the liquid solvent and the particles may nucleate and aggregate. There are many unclear points and open questions about the correlation between experimental conditions of LASiS, plume composition and size evolution of NPs. The answers for these questions are fundamental to better understand the growth process of nanoparticles and nanocrystals and to improve the engineering of nanoparticles production.
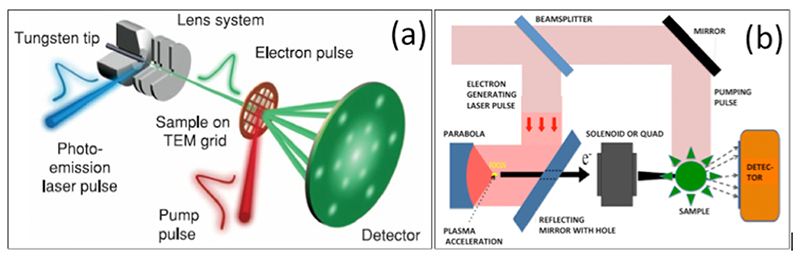
a) Sketch of a typical “conventional” Electron diffraction experiment; b) schematic setup for a laser-driven electron diffraction experiment [Image extracted from M. Gulde, Science 345, 200 (2014)]
The goal of this project is the generation of high-flux, high-energy laser-accelerated electrons with fs-scale temporal duration for performing Electron Diffraction experiments with improved electron parameters.
The project makes benefit of higher accelerating gradients (~TV/m), higher flux (~10^4 electrons per shot) and shorter pulse duration (25 fs at the source) that can be obtained with laser-plasma acceleration. Experiments are performed on different short-pulse laser facilities (e.g. our ALLS Laser and on the newly commissioned FORTH fs-laser (18 fs, 400 mJ, 10 Hz), manufactured by Amplitude). This project builds on preliminary and very promising experimental results related to this acceleration process that has already been achieved on the ALLS facility and published recently[10],[11],[12], achieving 23 keV electrons with 1.2 106 electrons per shot and tens of fs temporal duration. Besides testing “traditional” wakefield accelerated electrons, the project wants to explore a new acceleration regime, commonly called also “direct acceleration”, in which the polarization of the laser, used for the acceleration process, is modified with a specific waveplate. This allows having a strong longitudinal component on axis which allows boosting the acceleration process even further, and in particular for short pulse (
[1] Z. Ning, H. Tian, C. yuan, Y. Fu, H. Qin, L. Sun, H. Agren, Chem. Commun., 47, p. 1536, 2011
[2] A.M. Smith, S. Nie, Acc. Chem. Res., 43, p. 190, 2010
[3] A.P. Alivisatos, Nat. Mater., 2, p. 382, 2003
[4] V. Amendola, M. Meneghetti, J. phys. Chem. C, 113, p. 4277 (2009)
[5] A.S. Avilov, S.P. Gubin, M.A. Zaporozhets, Kristallografiya 58, 785 (2013)
[6] J. R. Dwyer et al.,, Philosophical transactions of the Royal Society A 364 (2006)
[7] R Srinivasan, V.A. Lobastov, C. Ruan, A. ZewailChim Acta 86 1763 (2003)
[8] S. Fourmaux, … V. Malka, A. Rousse and J. C. Kieffer Appl. Phys. Lett. 101 , 111106 (2012)
[9] B. Siwick, A.A. Green, C.T. Hebeisen, R.J. Miller, Chem. Phys. 299, 285 (2004)
[10] S. Payeur, S. Fourmaux, … F. Légaré, M. Piché, and J. C. Kieffer, Applied Physics Letters 101, 041105 (2012)
[11] S. Techert, F. Schotte,M. Wulff, Phys. Rev. Lett. 86, 2030 (2005)
[12] C. Varin, S. Payeur, … N. Thiré, T. Brabec, F. Legaré, J.C. Kieffer, M. Pichè, Applied Science 3, 70 (2013)